Transitioning from FDD to TDD has enhanced spectrum efficiency in the realm of 5G technology. However, this shift has introduced new timing challenges that need to be addressed to maintain network synchronization.
The landscape of telecommunications has been revolutionized by 5G technology, offering faster data speeds, reduced latency, and improved connectivity. However, the deployment of 5G networks necessitates substantial financial investments, particularly in spectrum licenses. Major mobile operators in the US have collectively spent close to $100 billion on mid-band spectrum for 5G.
Efficient utilization of spectrum is paramount to maximizing the ROI on these investments. Time and phase synchronization play a pivotal role in optimizing 5G spectrum utilization, focusing on technologies like Time Division Duplex (TDD), Carrier Aggregation (CA), and synchronization strategies supported by the O-RAN Alliance.
The Significance of Synchronization in 5G Networks
In previous mobile network generations, synchronization facilitated call handovers between base stations as users moved between cells. In the realm of 5G, synchronization is even more crucial, enabling the high capacity and low latency that users demand. For example, a 3 GB movie that takes 30 minutes to download on a 4G network can be downloaded in just 35 seconds on a 5G network.
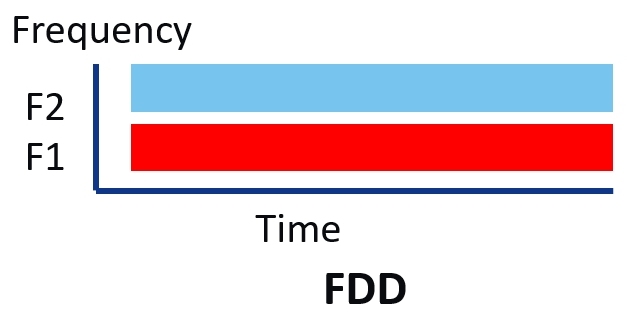
Figure 1. FDD separates uplink and downlink transmissions by frequency.
A 4G network predominantly operates in Frequency-Division Duplex (FDD) mode, utilizing paired frequency bands to facilitate simultaneous uplink and downlink transmission with a guard band in between. However, FDD’s fixed, symmetric 50/50 uplink/downlink ratio is suboptimal in terms of spectrum utilization (Figure 1).
Transitioning to Time-Division Duplex (TDD) in 5G
The introduction of Time-Division Duplex (TDD) mode in 5G offers enhanced flexibility and capacity. TDD requires only one band for both uplink and downlink, with a small guard period when switching between the two. The transmission is time-multiplexed into slots that can be dynamically allocated for uplink and downlink based on traffic demands.
TDD surpasses FDD in efficiency by enabling dynamic allocation of time slots, optimizing spectrum utilization (Figure 2). Furthermore, TDD is cost-effective as it eliminates the need for a diplexer to isolate transmission and reception. TDD is ideal for 5G features like massive Multiple Input Multiple Output (mMIMO), beamforming, and pre-coding techniques that enhance spectral efficiency and coverage reliability.
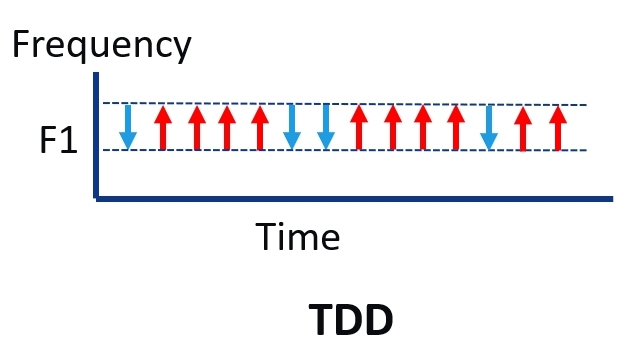
Figure 2. TDD mode uses time slots for uplink and downlink transmissions.
Synchronization in TDD
Effective time and phase synchronization are vital in TDD to prevent cross-slot interference between uplink and downlink transmissions. Base stations (referred to as remote units in 5G) must adhere to the same reference for downlink and uplink time slot assignments. Without synchronization, interference can be managed to some extent, but this necessitates stringent RF emission requirements, isolation distances, and larger guard bands, all of which compromise spectrum efficiency and escalate operational costs (Figure 3).
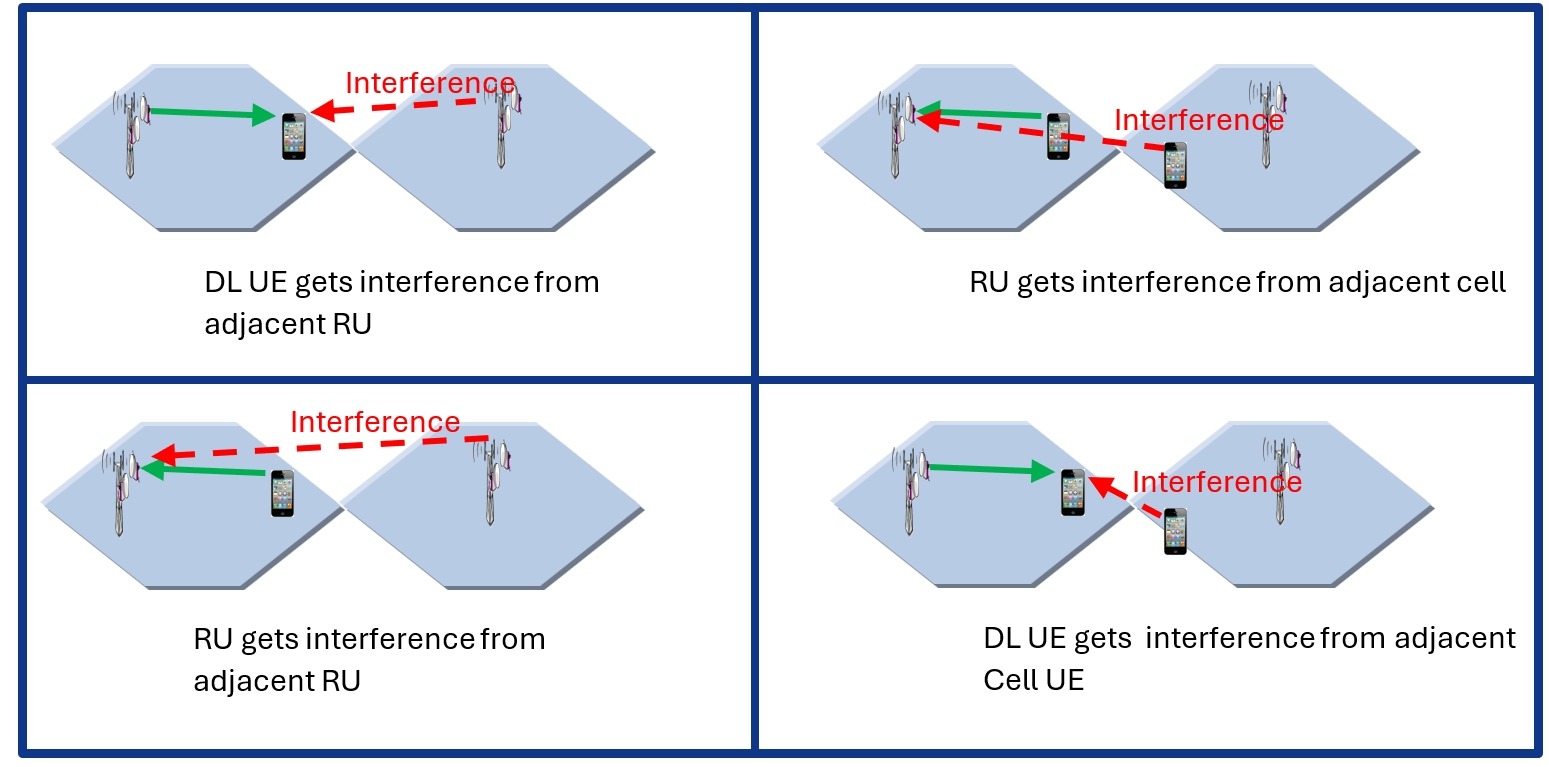
Figure 3. Interference cases arise from adjacent radios and cells.
The GSM Association (GSMA), a non-profit industry body representing mobile network operators globally, recommends that all TDD networks, whether LTE or 5G NR, operate in the same frequency range and within the same area for synchronization. It also calls for regulators to prioritize TDD synchronization to mitigate interference issues across national and international networks.
Carrier Aggregation in 5G
Carrier Aggregation (CA) is another key feature of 5G that maximizes spectrum utilization. CA combines multiple channels within the same FDD and TDD frequency bands or across different frequency bands to boost capacity and achieve higher data rates. This technology is essential for expanding coverage, reducing the need for additional cell sites, and cutting deployment expenses.
5G operates in two frequency ranges: FR1 (sub 6 GHz) and FR2 (mmWave, 24.25 GHz to 52.6 GHz). FR1 further segregates into low band (sub-1 GHz) and mid-band (1 GHz to 6 GHz). Low-frequency bands offer extensive coverage due to their deep indoor penetration. These bands are typically deployed in FDD mode, which exhibits stronger uplink signal strength compared to TDD. High-frequency bands in TDD mode deliver superior capacity and lower latency but suffer from weaker uplink signal strength compared to mid- and low-frequency bands. Mid-frequency bands in TDD mode strike a balance between coverage and capacity (Table 1).
Low-frequency band (FR1)-FDD |
Mid-frequency band (e.g., C-Band) (FR1)-FDD |
High-frequency band, mmWave-TDD |
|
Coverage | *** | ** | * |
Bandwidth | * | ** | *** |
Figure 4 illustrates that CA mandates specific bandwidth levels for time and phase synchronization. For instance, FR2 intra-band necessitates a maximum Time Alignment Error (TAE) of 130 ns, while FR1 intra-band contiguous and FR2 intra-band non-contiguous require a maximum TAE of 260 ns.
Synchronization in Open RAN 5G Networks
The O-RAN Alliance has emerged as a pivotal standard-setting body influencing the architecture of 5G networks. Established in 2018, the O-RAN Alliance aims to disaggregate the Radio Access Network (RAN) with open and standardized interfaces, mirroring the trend seen in data centers. This approach fosters innovation and reduces deployment costs by enabling mobile operators to mix and match interoperable components from diverse vendors.
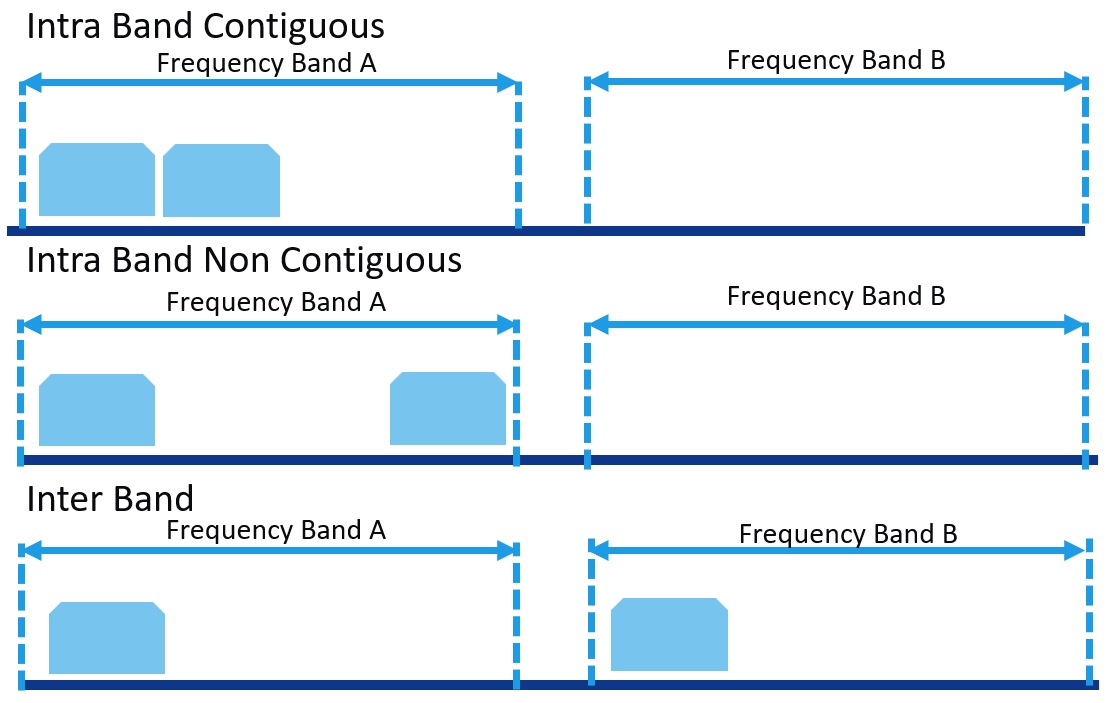
Figure 4. Carrier aggregation lets data travel in both intra-band and inter-band combinations, improving spectrum use.
Traditional cell sites comprised a Remote Radio Head (RRH), GNSS antenna, and a Baseband Unit (BBU) deployed at cell sites. In contrast, the architecture of 5G networks has been disaggregated into Radio Units (RUs), Distributed Units (DUs), and Centralized Units (CUs). Centralizing and sharing BBUs as DUs and CUs yield substantial operational expenditure (OpEx) savings in terms of power and cooling. Cell sites consume approximately 70% of the power energy in RAN. Sharing BBU processors in a centralized location also delivers noteworthy capital expenditure (CapEx) savings, as they were previously utilized at a fraction of their full capacity at cell sites. The software for RU, DU, and CU can be virtualized, containerized, and deployed on cost-effective general-purpose hardware (Figure 5).
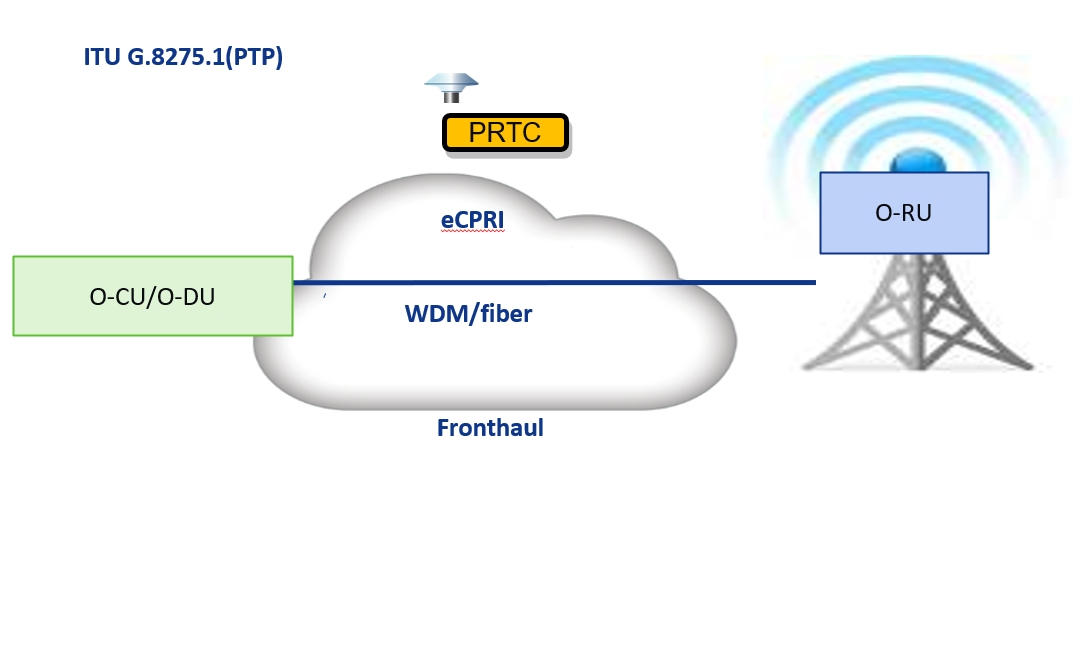
Figure 5. 5G Fronthaul uses a standardized eCPRI interface.
In previous mobile network generations, it was common practice to deploy a GNSS Primary Reference Time Clock (PRTC) locally at each cell site. Any updates, upgrades, or maintenance had to be carried out at each site. Moreover, most GNSS antenna receivers are accurate within ±100 ns, posing challenges for deploying key features like CA.
For 5G, the O-RAN Alliance advocates for network timing distribution as the preferred synchronization strategy, utilizing open and standardized ITU-T G.8275.1 and ITU-T G.8275.2 protocols. The ITU-T G.8275.1 PTP Telecom profile is implemented in the fronthaul network from a Primary Reference Time Clock Telecom Grandmaster (PRTC/T-GM) that synchronizes switches equipped with boundary clocks, DUs, and RUs.
Introducing a PRTC/T-GM in the fronthaul network aligns with the 5G disaggregated RAN architecture, centralizing timing functions in a single location for flexible and cost-effective sharing, maintenance, and upgrades. This approach eliminates the need for deploying a local PRTC/T-GM at each cell site and eliminates the necessity for more expensive oscillators at cell sites.
Microchip has conducted extensive synchronization, interoperability, and performance assessments with various vendors in the mobile network ecosystem. These tests, conducted during multi-vendor interoperability showcases by EANTC and IEEE ISPCS “plugfests,” ensure that Microchip’s products adhere to the highest performance and reliability standards.
Conclusion
Time and phase synchronization are crucial aspects of 5G networks, enabling technologies like TDD and CA to optimize spectrum utilization and reduce deployment expenses. The vision of the O-RAN Alliance for a disaggregated RAN with open and standardized interfaces underscores the importance of synchronization in contemporary network architectures.
By implementing robust synchronization strategies and leveraging advanced timing solutions, mobile operators can ensure efficient utilization of the 5G spectrum, ultimately enhancing network performance and cutting operational costs.
Related content
Explore EE World’s assortment of timing articles for further insights into 5G network timing.